Abstract
The development of environmentally friendly organic synthetic reactions is an important research issue that is fundamental to the realization of a sustainable society. In our laboratory, we mainly use transition metal complexes as catalysts for the development of environmentally friendly organic synthetic reactions. In particular, we are working toward the development of oxidation reactions using alkenes and other hydrocarbons as raw materials, and molecular oxygen as a terminal oxidant. In addition, we are studying the creation and reactivity of new transition metal complexes that can catalyze those synthetic organic reactions. We are also developing functional organometallic compounds that target the substructures of nucleic acids (DNA and RNA).
Current Research Subjects
1. Development of Catalytic Oxidations of Alkenes Using Molecular Oxygen
2. Development of Catalytic Dehydrogenations of Saturated Carboxylic Acids Using Molecular Oxygen
3. Reactivity of Transition-Metal Complexes for Development of Catalytic Oxygenations
4. Development of Functional Organometallic Compounds (G4 Ligands) Targeting the Guanine Quadruplex (G4)
1. Development of Catalytic Oxidations of Alkenes Using Molecular Oxygen
1-1. Synthesis of Aldehydes from Terminal Alkenes (Anti-Markovnikov Wacker-type Oxidation)
If the terminal position of terminal alkenes can be selectively oxidized under mild conditions using molecular oxygen, it can be an efficient method for aldehyde synthesis from terminal alkenes (Anti-Markovnikov Wacker-type oxidation). This synthetic method is superior in principle to hydroformylation, the current industrial process for manufacturing aldehydes from alkenes, because it does not use high pressure synthesis gas (a mixture of carbon monoxide and hydrogen) as hydroformylation does, and it is safer.
We have found a palladium/copper-catalyzed synthesis of arylacetaldehydes from aromatic alkenes using oxygen as a terminal oxidant. In this reaction, the addition of a small amount of an electron-deficient cyclic alkene, such as maleimide, has made it possible to achieve a reaction under oxygen at ambient pressure, which could not be achieved in the past.[1-3]
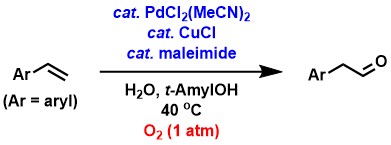
In the synthesis of aldehydes from aliphatic terminal alkenes, the use
of p-benzoquinone (BQ) in addition to a palladium/copper catalyst was found
to give products with good aldehyde selectivity.[2-4] Wacker-type oxidation of simple aliphatic terminal alkenes usually produces
exclusively ketones with little or no aldehydes, but we have found that
the above catalyst system and the slow addition of alkenes and water to
the reaction solution significantly improve the selectivity of aldehydes
over ketones.
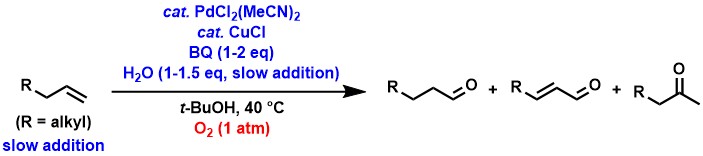
Currently, we are working to further improve aldehyde selectivity and catalytic
activity in these reactions.
We also found that aldehyde synthesis proceeds from bulky 1,1-disubstituted alkenes.[5] Although only a few examples of Wacker-type oxidation from 1,1-disubstituted alkenes are known, we have found that cyclic hemiacetals can be obtained via aldehyde formation by applying a palladium complex / NO / BQ catalyst system using 3-methyl-3-buten-1-ols as substrates and oxygen as an oxidant at ambient pressure, respectively.
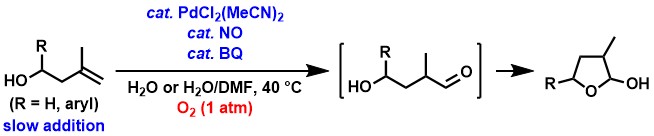
1-2. Synthesis of Terminal Acetals from Terminal Alkenes (Anti-Markovnikov
Oxidative Acetalization)
As a related oxidation reaction to the aldehyde synthesis described above, we have also developed the synthesis of terminal acetals from terminal alkenes.[2,3,6] Terminal acetals are the protected compounds of aldehydes and are useful in synthetic chemistry. The reaction proceeds efficiently by using aromatic alkenes, allyl ethers, and 1,5-dienes as substrates and BQ as an oxidant.

Further developing the above acetal synthesis, we have also found a catalyst
system that can use oxygen at ambient pressure instead of BQ as an oxidant.[2,3,7] The addition of a catalytic amount of methoxy-p-benzoquinone (MeOBQ) is key to this reaction. Kinetic experiments show
that MeOBQ acts as a ligand for palladium to accelerate the reaction, making
it an efficient catalytic system.
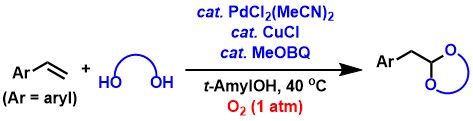
Terminal acetal synthesis reactions from aliphatic terminal alkenes are
very difficult to achieve, as is the case with aldehyde synthesis reactions.
We have succeeded in efficiently carrying out this reaction by applying
reaction conditions similar to those of aldehyde synthesis from aliphatic
terminal alkenes.[2,3,8]
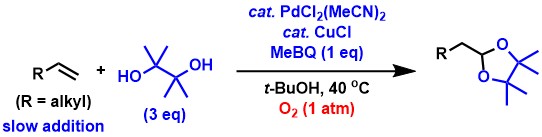
Currently, we are working to further improve terminal selectivity and catalytic
activity in these reactions and to expand the scope of substrates.
References
1. Nakaoka, S.; Murakami, Y.; Kataoka, Y.; Ura, Y.*, Maleimide-assisted
Anti-Markovnikov Wacker-type Oxidation of Vinylarenes Using Molecular Oxygen
as a Terminal Oxidant, Chem. Commun. 2016, 52, 335-338.
2. Ura, Y.*, Palladium-Catalyzed Anti-Markovnikov Oxidation of Aromatic
and Aliphatic Alkenes to Terminal Acetals and Aldehydes, Synthesis 2021,
53, 848-860.
3. Ura, Y.*, Realization of Anti-Markovnikov Selectivity in Pd-Catalyzed
Oxidative Acetalization and Wacker-Type Oxidation of Terminal Alkenes,
Chem. Rec. 2021, 21, 3458-3469.
4. Komori, S.; Yamaguchi, Y.; Murakami, Y.; Kataoka, Y.; Ura, Y.*, Palladium/Copper‐catalyzed
Oxidation of Aliphatic Terminal Alkenes to Aldehydes Assisted by p‐Benzoquinone,
ChemCatChem 2020, 12, 3946–3955.
5. Tanaka, R.; Komori, S.; Shimizu, Y.; Kataoka, Y.; Ura, Y.*, Synthesis
of 2-hydroxytetrahydrofurans by Wacker-type oxidation of 1,1-disubstituted
alkenes, Org. Biomol. Chem. 2022, 20, 570–574.
6. Yamamoto, M.; Nakaoka, S.; Ura, Y.*; Kataoka, Y., Palladium-catalyzed
Synthesis of Terminal Acetals via Highly Selective Anti-Markovnikov Nucleophilic
Attack of Pinacol on Vinylarenes, Allyl Ethers, and 1,5-Dienes, Chem. Commun.
2012, 48, 1165-1167.
7. Matsumura, S.; Sato, R.; Nakaoka, S.; Yokotani, W.; Murakami, Y.; Kataoka,
Y.; Ura, Y.*, Palladium-catalyzed Aerobic Synthesis of Terminal Acetals
from Vinylarenes Assisted by pi-Acceptor Ligands, ChemCatChem 2017, 9,
751-757.
8. Komori, S.; Yamaguchi, Y.; Kataoka, Y.; Ura, Y.*, Palladium-catalyzed
Aerobic anti-Markovnikov Oxidation of Aliphatic Alkenes to Terminal Acetals,
J. Org. Chem. 2019, 84, 3093–3099.
2. Development of Catalytic Dehydrogenations of Saturated Carboxylic Acids Using Molecular Oxygen
α,β-Unsaturated carboxylic acids are an important group of compounds used as synthetic intermediates in organic synthesis because they exhibit a wide variety of reactivity, including conjugate addition. Industrially, for example, acrylic acid is used as a raw material for sodium polyacrylate (superabsorbent polymer) and acrylic esters (used in paints, textile chemicals and other products through copolymerization). On the other hand, long-chain saturated carboxylic acids and short-chain saturated carboxylic acids are renewable raw materials obtained from biomass through hydrolysis of fats and oils, and microbial metabolism of organic compounds, respectively. Therefore, if it becomes possible to efficiently synthesize α,β-unsaturated carboxylic acids from saturated carboxylic acids in an environmentally low-impact manner, it will be an excellent production method for α,β-unsaturated carboxylic acids from a long-term perspective.
We have discovered a method for the synthesis of α,β-unsaturated carboxylic acids by dehydrogenation of saturated carboxylic acids using a palladium acetate/pyridine catalyst system and safe, cheap, and abundant oxygen as an oxidant.[1] When propionic acid was used as a feedstock, a corresponding dehydrogenation product, acrylic acid, and a conjugate addition product of propionic acid to acrylic acid, were obtained, respectively. The conjugate addition product can be decomposed into acrylic acid and propionic acid by heating. Both palladium acetate and pyridine were essential, and in their absence the reaction either did not proceed at all or the amounts of products obtained were greatly reduced.

Besides propionic acid, the reaction could be applied to saturated carboxylic
acids with various hydrocarbon chains, including longer linear and branched
structures.
Currently, we are studying ways to dramatically improve catalytic activity.
References
1. Shibatani, A.; Kataoka, Y.; Ura, Y.*, Palladium-Catalyzed Aerobic α,β-Dehydrogenation
of Carboxylic Acids, Asian J. Org. Chem. 2021, 10, 3285–3289.
3. Reactivity of Transition-Metal Complexes for Development of Catalytic Oxygenations
If molecular oxygen can be used as an oxidant to efficiently and selectively synthesize oxygen-containing organic compounds such as alcohols and aldehydes from hydrocarbons, these could be important synthetic reactions both industrially and at the laboratory level.
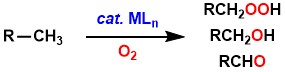
Toward the development of such catalytic oxygenation reactions, our laboratory
is studying the reactivity of transition metal complexes having hydrocarbon
ligands with oxygen. We have found that the stoichiometric reaction of
benzylpalladium complexes with oxygen is significantly accelerated by the
addition of anion sources such as n-Bu4NX and Brønsted acid.[1] This reaction yields oxygenated products (benzaldehyde, benzyl hydroperoxide,
benzyl alcohol) derived from the benzyl ligands. The absence/presence of
protons changes the main product and the reaction mechanism.
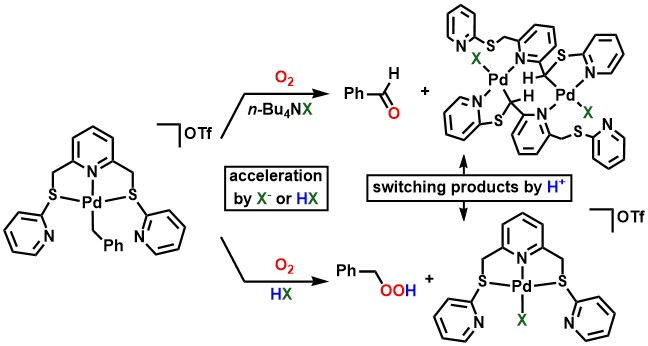
Based on the result that the presence of a proton accelerates the oxygenation
of the benzyl ligand, we wondered if the oxygenation could be further accelerated
by placing the Brønsted acid moiety close to the metal atom, and focused
on secondary phosphine oxides (SPOs) as such ligands. SPOs are tautomeric
with phosphinous acids, which can coordinate to the metal at the phosphorus
atom and place a Brønsted acid moiety right next to it.
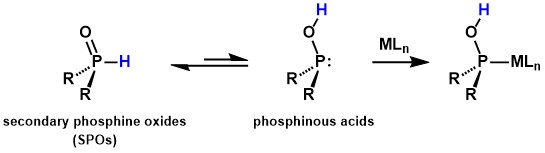
When a benzyl palladium complex [PdBn(Cl)(cod)] (cod = 1,5-cyclooctadiene)
was reacted with diphenylphosphine oxide as a SPO under an oxygen atmosphere,
oxygenation of the benzyl ligand proceeded rapidly at room temperature
in 30 minutes, producing benzyl hydroperoxide in high yield.[2] In the absence of SPOs, the oxygenation of the benzyl ligand did not proceed
at all, indicating that SPOs accelerate the oxygenation. When the same
reaction was performed with a smaller amount of oxygen (0.5 equiv. of the
complex), benzyl alcohol was selectively formed instead of benzyl hydroperoxide.[2]
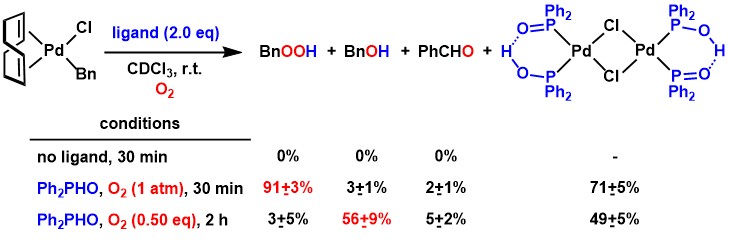
Based on some experimental results, we believe that the proton of the phosphinous
acid ligand promotes the reaction of palladium with oxygen as follows.
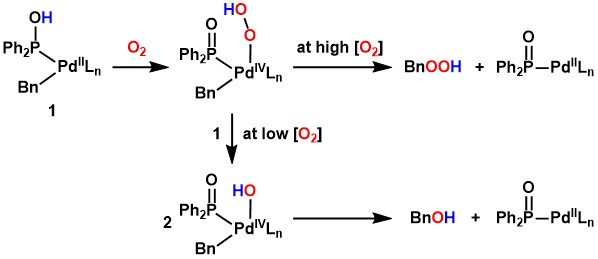
Oxygenation of hydrocarbon ligands such as benzyl ligands can be regarded
as a key step to realize catalytic oxygenation of difficult hydrocarbons,
and the above results will provide important knowledge for the realization
of such catalytic reactions.[1-3]
Currently, we are investigating more detailed reaction mechanisms for the
oxygenation and are conducting research to develop catalytic oxygenation
reactions of hydrocarbons.
References
1. Shimokawa, R.; Kawada, Y.; Hayashi, M.; Kataoka, Y.; Ura, Y.*, Oxygenation
of a Benzyl Ligand in SNS-Palladium Complexes with O2: Acceleration by
Anions or Brønsted Acids, Dalton Trans. 2016, 45, 16112–16116.
2. Oka, S.; Shigehiro, Y.; Kataoka, Y.; Ura, Y.*, Secondary Phosphine Oxide-triggered
Selective Oxygenation of a Benzyl Ligand on Palladium, Chem. Commun. 2020,
56, 12977–12980.
3. Shigehiro, Y.; Miya, K.; Shibai, R.; Kataoka, Y.; Ura, Y.*, Synthesis
of Pd-NNP Phosphoryl Mononuclear and Phosphinous Acid-Phosphoryl-Bridged
Dinuclear Complexes and Ambient Light-Promoted Oxygenation of Benzyl Ligands,
Organometallics 2022, 41, 2810–2821.
4. Development of Functional Organometallic Compounds (G4 Ligands) Targeting the Guanine Quadruplex (G4)
G-quadruplexes, called by G4s, are known as one of the higher-order structures of nucleic acids in guanine-rich single-strand sequences. G4s play a regulatory role in various biological phenomena, including the apoptosis of cancer cells and the regulation of transcription and translation. Recently, the formation of G4s in the corona-virus genome has been reported to potential treatments for COVID-19. For the above reasons, G4s are considered a "new regulatory factor in biological phenomena", and small molecules that stabilize G4 (G4 ligands) seen as promising "drug seeds" and "molecular probes".
In our laboratory, we are focusing on the development of functional organometallic compounds (G4 ligands) targeting G4. Our goal is to harness these ligands for treating G4-related diseases, initiating biochemical reactions in the body through G4, and selectively visualizing target molecules.
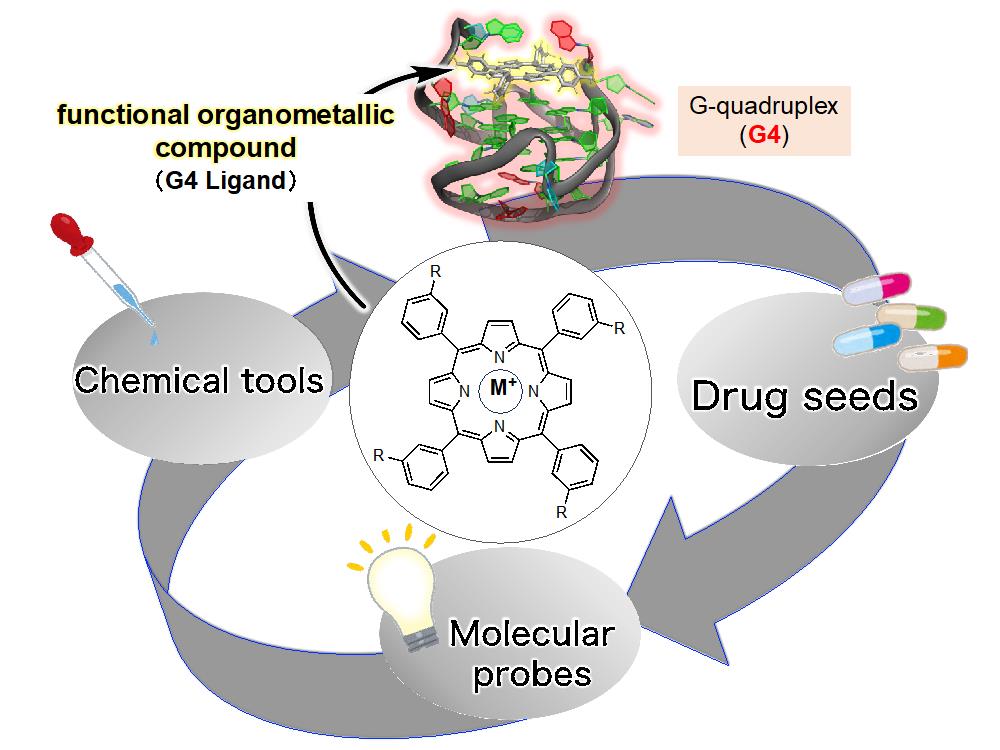